Fundamental (Single Cell) Limits to Minimally Invasive Surgery and Biodiagnostics
This group is taking advantage of new insights into strongly driven phase transitions based on the very first “molecular movies” in which the relative atomic motions were captured faster than collisions could blur out the details of atomic motions moving relative to one another (Siwick et al Science 2003). This work showed that for sufficient superheating rates, the phase transition propagates through a process known as homogeneous nucleation (melting from the inside out) rather than the normal everyday occurrence of heterogeneous nucleation (melting from the outside in). Most important, for sufficiently fast superheating, the nucleation sites are confined solely to nearest neighbor disordering or only approximately 10 atoms per nucleation site (single unit cell). This singular observation may seem of only esoteric interest, however, it has to be realized that explosive nucleation growth and the resulting cavitation induced shock waves is what causes massive collateral damage in laser surgery - till this work. By using short enough pulses, but not so short to lead to peak powers that result in plasma formation and ionizing radiation effects, it is possible to effectively completely eliminate nucleation growth and cavitation problems in ablating material. This new insight was explicitly exploited in the development of a new class of solid state mid IR laser systems that are tuned to the vibrational stretch of water, which has an extremely strong absorption band, absorptivity in excess of 104 cm-1. Tissue is made of 60-70% water such that the interstitial water is energized by the absorption of the IR that effectively goes directly into translational motion through the strong hydrogen bonding within the water. The water acts like a propellant to drive everything within the absorption volume that is above the phase transition into the gas phase faster than even collisional exchange with the constituent protein matrix and without nucleation formation and cavitation induced shock waves. The extremely large amplitude strain fields (sound waves) generated by the ablation process are in the 100-10 GHz range, which are so strongly attenuated at this high frequency that even this normal loss mechanism goes into driving the ablation.
The laser system specifically designed to exploit this new atomic insight is referred to as the Picosecond InfraRed Laser (PIRL) scalpel.
Basically, the laser system pulse parameters were developed to match the impulse response function of liquid water under strong enough superheating to ensure that the laser driven phase transition (liquid to gas) and ablation forces occurs with complete energy confinement. There is no acoustic or thermal transport out of the excited volume that would otherwise cause collateral damage to surrounding tissue. The ultrafast nature of the superheating ensures the phase transition occurs at the spinoidal decomposition point in which the nucleation sites are only a few 10 nm to 100 nm in diameter (pulse width dependent), as described above. The ensuing phase explosion occurs in the homogeneous nucleation limit where the degree of homogeneity extends uniformly throughout the lattice. This mechanism effectively cuts tissue with no collateral damage to adjacent tissue with single cell precision.
It is extremely important that the laser pulses used to achieve this fundamental limit to the phase transition dynamics are short enough to achieve this goal but long enough in duration to avoid peak powers that will lead to multiphoton ionization and plasma formation. The group did some earlier studies on laser surgery with femtosecond laser pulses (multiphoton ionization and plasma formation). We found fantastically sharp boundaries for the laser cutting but retarded healing. The net effect is a massive dose of ionizing radiation that kills adjacent cells so cleanly that normal healing processes are not initiated by the damage. This observation pointed out the serious problem in using such a highly ionizing source. (If you don’t like ionizing radiation effects from x-rays, think about removing a pound of flesh by such a highly ionizing radiation source.)
We knew at the time that PIRL would represent the fundamental upper limit to minimal invasive laser surgery. Water has a much lower boiling point in its phase diagram relative to all other local parameters for the constituent tissue matrix. By selectively targeting water, we could drive the phase transition fast enough to avoid explosive nucleation and cavitation induced shock waves. Fast enough to avoid any energy transport and damage to surrounding tissue. The ablation physics are such that the material removal is actually faster than thermal transport and even acoustic transport of energy out of the excited zone. In addition, the whole process occurs on timescales faster than collisional energy exchange of energy of the highly excited water and constituent proteins. These effects along with the extremely short penetration depth of < 1 micron (perfectly matched to the thickness of a single cell) ensure that PIRL would leave the least amount of collateral damage fundamentally possible. However, we did not expect that the damage would be so minimal that there would be essential no scar tissue formation. The healing process is triggered but it only involves the adjacent cells without any highly entangled fibroblast formation from damage zones nearly 1mm from the cut. This mottled formation of fibroblasts is what we call scar tissue. With PIRL, we observed perfect healing with far less protein signaling for healing pathways in comparison to the gold standard scalpel. PIRL represents the first means to execute surgery without scar tissue formation. It is the scar tissue buildup and blocked restructuring of tissue that leads to loss in function for all surgeries to date. One can well imagine that the ability to cut tissue without scar tissue formation will have many applications in plastic/cosmetic surgery. (Right now we can make beautiful mice and are moving forward to tissue models closer to humans). However, the real tangible improvements in surgical outcomes will be seen in procedures requiring high precision and the need to avoid scar tissue formation. Important applications in neurosurgery, vocal cord repair, microcholea implants for hearing, and restructuring of critical vasculature are readily envisaged.
This group is charged with advancing the laser technology, beam delivery, and surgical tools to take PIRL into the operating room (OR). There is one application that has now passed clinical trials for a procedure that is done on >10M people per year world wide. This is just one application. We are also exploring robotics as the accuracy of PIRL is well beyond the ability of even the most skilled surgeon. This development will eventually lead to the adoption of the PIRL scalpel for all point of entry surgical incisions with new means already in development to go well beyond present state of the art in minimally invasive surgery.
In addition to surgical applications, PIRL ejects intact proteins into the gas phase completely intact and in their native conformation, as confirmed by mass spectral analysis of the ablation process. This observation may turn out to be the most important of all. We now have a matrix independent means to eject entire proteins into the gas phase intact, as an essential step for using mass spectrometry for protein detection. Mass spectrometry (MS) is based on charged particle detection, which in principle can attain single molecule detection limits. There are methods such as electrospray and MALDI that can also introduce proteins into the gas phase. However, the ion collection efficiency is typically less than 10-5 considering sample collection, fractionization, introduction losses into the MS and the ensuing ionization physics (coulomb explosion of charged droplets in the electrospray case and proton exchange under super heated conditions of MALDI). The multiple source terms of loss lead to highly nonlinear signals and signal suppression of multiple component systems. MS analysis presently is the most sensitive method for biodiagnostics (without need of labels) but nevertheless requires purification of sample and extremely time intensive preparation steps. PIRL opens up the door to in situ mass spectrometry based biodiagnostics.
The group is developing a new imaging mass spec system specifically designed to take advantage of the unique attributes of PIRL in quantitative introduction of proteins into the gas phase without signal suppression. This is an exciting development as in principle it should be possible to approach nearly 100% ion collection efficiencies with proper ion optics coupled to the PIRL ablation process.
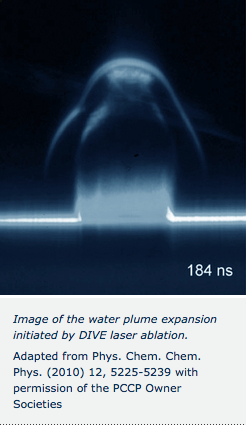
The image of the water plume expansion demonstrates the uniform forces involved in selectively exciting water. Laser ablation processes leading to nucleation bubbles, cavitation, and shock wave formation lead to huge shear forces that eject material into “\( 4 \pi \)”, i.e., in all directions like a mushroom cloud. The very fact that the PIRL driven ablation looks like a highly directed “laser beam” of matter proves the forces are all uniformly along the surface normal, tracking the temperature gradient impulsively imposed in the sample.
The group is working to advance PIRL and Mass spectrometry (referred to as Desorption by Impulsive Vibrational Excitation: DIVE-MS) towards the fundamental limit of single protein detection for biodiagnostics. Such a development will speed up biodiagnostics by orders of magnitude (less time required for sample preparation, much less sample required) and can be used in tandem with laser surgery to provide molecular feedback in real time to surgeons to guide surgery. Surgeons currently rely solely on tactile and visual feedback of the operating field. Soon they will have a new sense to guide them – “smell”. The PIRL-DIVE-MS is effectively a molecular nose that will give surgeons an intact molecular signature of the tissue being excised – a veritable molecular bar code to tell them what tissue they are cutting. This feature of PIRL ensures the right tissue is removed. Even if PIRL cuts at single cell precision, it is hardly minimally invasive if the wrong tissue is removed or cancerous tissue/borders are not removed sufficiently. It is the combination of single cell precision in excising tissue and molecular level identification that has allowed PIRL technology to truly achieve the fundamental single cell limit to minimally invasive surgery.
All of these potential applications came from the fundamental research program directed at achieving an atomic level view of structural transitions – to realize the Chemists’ collective gedanken experiment. It is a testimony to the importance of basic research. One can never predict where new insights will lead, just that the views will be wonderful to behold.