The experimental studies of the ultrafast phenomena performed in the research group require table top laser systems that are at the frontier of today’s laser technology. We try to push limits in terms of ultrashort pulse’s peak power, duration and tunability with simultaneous control of its optical phase and amplitude. Since the lasers are used in actual experiments to induce nonlinear effects and probe fine features of the quantum dynamics, special care is taken to ensure their short and long term stability to increase signal to noise ratio and avoid measuring artifacts.
Over the years, the group has accumulated considerable laser expertise. A number of developments currently in use in commercial lasers, from electro-optics to HV high speed drivers used in pockels cells for regenerative amplifiers, trace their roots to our group. Essentially all electro-optic crystals used for switching and amplification came from our group (BBO, all biaxial crystals such as KTP, KTA etc.) A number of laser systems and optical systems have been commercialized. You have a 70% chance of flying on an aircraft in which the many kms of aerospace wiring was marked by our laser systems to avoid wiring mistakes and to reduce risk of on board electrical fires (Lumonics circa 2000). Intel has used our lasers in high density packaging (Lumonics circa 2000). The group has also developed the first laser system to achieve the long held promise of attaining the fundamental (single cell) limit to minimally invasive surgery – and first method capable of surgery without scar tissue formation - the Picosecond InfraRed Laser (PIRL, LMI Inc. 2020).
The current solid state laser development is directed towards compact, robust, femtosecond laser systems capable of serving as the optical engine for femtosecond electron diffraction studies and spatial imaging. The ultrabright electron sources developed by the group require only microjoule level injection pulses to create the electron pulses. Similarly, sample excitation conditions need to stay within 1-photon excitation limits that again require 100 nanojule to 1 microjoule pulse energies for typical focusing conditions. There is no need for the millijoule class, very expensive, regeneratively amplified femtosecond systems currently sold commercially for this important class of experiments. The group has build up a fs fibre oscillator based on the work of Frank Wise’s group at Cornell and developed a large core fibre amplifier that provides pulse energies in the required energy class with 100 fs time resolution for the fundamental. This fs laser source is extremely stable, much more robust than regeneratively amplified systems requiring multiple lasers for pumping and is a fraction of the cost.
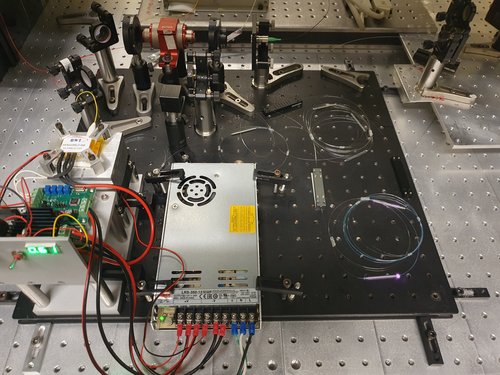
The image shows an all fibre amplifier built recently. The Yb doped fibre amplifier is used to amplify the pulsed laser beam at 130 mW to higher powers (several Watts). The laser pulses are stretched in time using a long fibre stretcher to reduce the pulse intensity. The pulses are then amplified using a Yb doped 10 micron core fibre. The doped fibre is pumped using a high power (27 Watts) diode laser. The residual pump is filtered out and only the amplified pulses come out of the amplifier. This fibre amplifier is compact, requires no maintenance, is resistant to temperature variations of the environment and it is relatively cheap.
This work aims to make atomically resolved reaction dynamics available to all groups by providing simple cost effective solutions to the femtosecond laser front end. Similarly, important applications are anticipated in medical application involving spatial imaging.
This technical expertise in laser source development has enabled the group to explore new domains, to go beyond the constraints of commercially available systems. In fact, the major emphasis is to develop new lasers to do experiments not possible with commercial systems. We decide which experiments are of interest – not the laser.